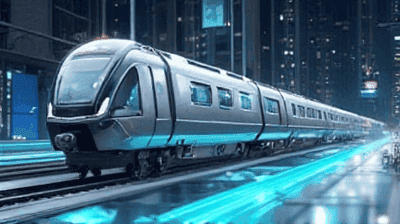
Imagine a world where energy loss during power transmission is virtually eliminated, where trains float effortlessly above their tracks, gliding silently at incredible speeds. This futuristic vision is not just a figment of science fiction; it is the promise of superconductors. These extraordinary materials exhibit a phenomenon known as superconductivity, allowing them to conduct electricity without resistance when cooled to extremely low temperatures.
What Are Superconductors?
Superconductors are materials that can conduct electric current with zero electrical resistance. This property emerges below a critical temperature unique to each material. As the temperature decreases and reaches this critical point, superconductors undergo a phase transition into a superconducting state.
Types of Superconductors
Superconductors can be categorized into two main types:
Type I Superconductors: These materials exhibit complete expulsion of magnetic fields when cooled below their critical temperature, a phenomenon known as the Meissner effect. Type I superconductors are typically pure elemental metals, like lead and mercury, and have relatively low critical temperatures.
Type II Superconductors: These are generally complex compounds and alloys that allow magnetic fields to penetrate through them in quantized vortices. They can handle much higher temperatures and magnetic fields compared to Type I superconductors, making them more suitable for practical applications.
A Brief History of Superconductivity
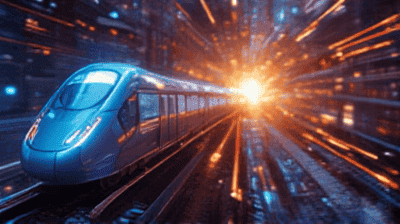
The discovery of superconductivity goes back over a century, marking a significant milestone in the field of material science.
Early Discoveries
1911: The phenomenon of superconductivity was first discovered by Dutch physicist Heike Kamerlingh Onnes while experimenting with mercury at cryogenic temperatures. He observed that mercury's electrical resistance dropped to zero at approximately 4.2 Kelvin, earning him the Nobel Prize in Physics in 1913.
1933: The Meissner effect was discovered by Walther Meissner and Robert Ochsenfeld, who found that superconductors could repel magnetic fields, leading to the understanding that superconductivity involves more than just zero resistance.
Advancements and High-Temperature Superconductors
The field of superconductivity saw a leap forward in the 1980s:
1986: Scientists Karl Müller and Johann Bednorz discovered a class of materials known as cuprates, which exhibited superconductivity at temperatures significantly higher than previously known superconductors. This discovery paved the way for superconductors that operate above the boiling point of liquid nitrogen (77 Kelvin).
2000s and Beyond: Research continues to find new superconducting materials, including iron-based superconductors and truly room-temperature superconductors, which promise to revolutionize various technological fields.
The Physics of Superconductivity
Understanding why superconductors behave the way they do requires diving into the realm of quantum mechanics and condensed matter physics.
Cooper Pairs and the BCS Theory
The foundation of conventional superconductivity is described by the Bardeen-Cooper-Schrieffer (BCS) theory, proposed in 1957. According to this theory:
Cooper Pairs: At low temperatures, electrons near the Fermi surface can form pairs, known as Cooper pairs, which allow them to move through the lattice structure of the material without scattering, thus avoiding resistance.
Energy Gap: Below the critical temperature, an energy gap opens up, preventing thermal excitations from breaking these Cooper pairs apart. This gap is crucial in maintaining superconductivity.
The Meissner Effect
The Meissner effect is a key characteristic of superconductors, distinguishing them from perfect conductors. When a material becomes superconducting, it expels external magnetic fields from its interior, resulting in magnetic field lines bending around the superconductor. This property plays a vital role in applications like maglev trains.
Applications of Superconductors
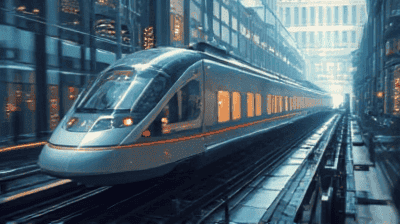
The unique properties of superconductors lend themselves to a variety of applications across multiple fields. Here are some of the most promising uses:
Energy Transmission
One of the most significant advantages of superconductors is their ability to transmit electricity with zero resistance. This can lead to enormous efficiencies in power grids:
Superconducting Power Cables: These cables can carry significantly higher currents than traditional copper wires, allowing for more efficient power distribution over long distances. By reducing energy loss, these cables can help improve the overall efficiency of energy systems.
Energy Storage: Superconducting magnetic energy storage (SMES) systems utilize inductors made of superconducting materials to store energy in the magnetic field. These systems can discharge energy rapidly, making them ideal for stabilizing power grids and providing backup energy.
Transportation: Maglev Trains
Maglev (magnetic levitation) trains utilize superconductors to achieve frictionless travel:
Levitation Mechanism: Superconductors exhibit the Meissner effect, which allows them to repel magnetic fields and effectively create lift. This enables trains to float above the tracks, eliminating friction and allowing for smooth movement.
Speed and Efficiency: Maglev trains can travel at speeds exceeding 300 miles per hour (483 kilometers per hour) while consuming less energy than traditional train systems. With developments in superconducting materials, future maglev trains may reach even greater speeds.
Medical Technology
Superconductors also play a critical role in medical imaging technologies:
Magnetic Resonance Imaging (MRI): Superconducting magnets are used in MRI machines, providing strong and stable magnetic fields essential for high-quality imaging. The use of superconductors enhances the resolution and clarity of medical scans.
Magnetoencephalography (MEG): This technique, utilized to measure the magnetic fields produced by neural activity in the brain, employs superconducting materials in devices like superconducting quantum interference devices (SQUIDs).
Scientific Research
Superconductors enable some of the most advanced scientific experiments:
Particle Accelerators: Superconducting magnets are critical in particle accelerators, such as the Large Hadron Collider (LHC), where they steer particles at nearly the speed of light. The high efficiency and strength of superconducting magnets make them indispensable in research.
Quantum Computing: Research into superconducting qubits, which leverage the unique properties of superconductors, is at the forefront of quantum computing advancements. These qubits can perform operations at high speeds and with minimal errors.
Future Innovations and Challenges
As researchers continue to explore the potential of superconductors, several challenges and areas of innovation are emerging.
Room-Temperature Superconductors
The quest for room-temperature superconductors has been a long-standing goal in material science. The discovery of such materials would revolutionize energy systems, transportation, and technology. Recent claims of achieving superconductivity at higher temperatures, under extreme pressures, have sparked excitement, but practical applications remain elusive.
Scalability and Cost
While superconductors hold immense potential, several practical issues must be addressed:
Manufacturing Costs: The cost of producing superconducting materials can be prohibitively high, hindering widespread adoption. Efforts are underway to develop cheaper manufacturing processes and materials.
Cooling Requirements: Most superconductors require cryogenic cooling, which entails additional costs and technological challenges. Finding ways to maintain superconductivity at higher temperatures or using alternative cooling methods is crucial for practical applications.
Integration into Existing Infrastructure
Integrating superconducting technology into current energy and transportation systems poses its own set of challenges. Transitioning from traditional systems to superconducting alternatives requires comprehensive planning, testing, and investment.
Conclusion
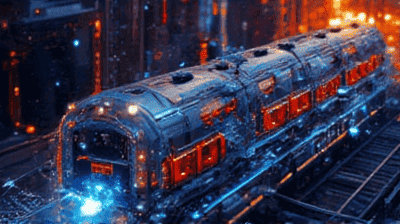
Superconductors represent one of the most exciting frontiers in modern science and technology. Their unique ability to conduct electricity without resistance holds the key to transforming how we generate, transmit, and utilize energy. Applications in transportation, medical technology, and scientific research demonstrate just a fraction of their potential.
As scientists continue to unravel the mysteries of superconductivity, the dream of a future powered by zero resistance energy and levitating trains may one day become a reality. By overcoming the current challenges and fostering innovation, superconductors have the potential to reshape our world, leading us into a new era of technological advancement and sustainability.